GPS satellite navigation is now ubiquitous in vehicles or on phones. But how do these devices know where you are? And how do geodetic instruments increase the precision enough to measure tectonic plate velocities of one millimeter per year or less?
The Global Positioning System (GPS) was created by the US Department of Defense but ultimately made available for public use. There are other such satellite constellations, including Russia’s GLONASS and the European Union’s Galileo system. The general name for these systems is Global Navigation Satellite System (GNSS), but for simplicity, we will refer to GPS here as each functions similarly.
GPS fundamentally consists of three components: satellites, ground stations, and receivers. There are currently 31 GPS satellites that actively orbit the Earth, with at least 24 required for global coverage. The position of each satellite must be precisely known in order for the system to work, so a network of ground stations is used to track their orbits. Finally, the receiver in your device records the signals from each satellite in the sky and uses that information to calculate your position on Earth.
Each satellite is equipped with an atomic clock to precisely and accurately measure the time, which is included in the signal it broadcasts to receivers along with the satellite’s current position. Based on the difference between the time that the signal was sent and the time that it was received, you can calculate the distance between the receiver and the satellite.
GPS receivers use a process called “trilateration” to compute their location based on the distance from each satellite. If you know your distance from one point in space, you could be located anywhere on a sphere around the point with a radius of that distance. That information alone is not very useful in determining your location, but if you know your distances from two different points, you can draw spheres around each of those points and see where they intersect.
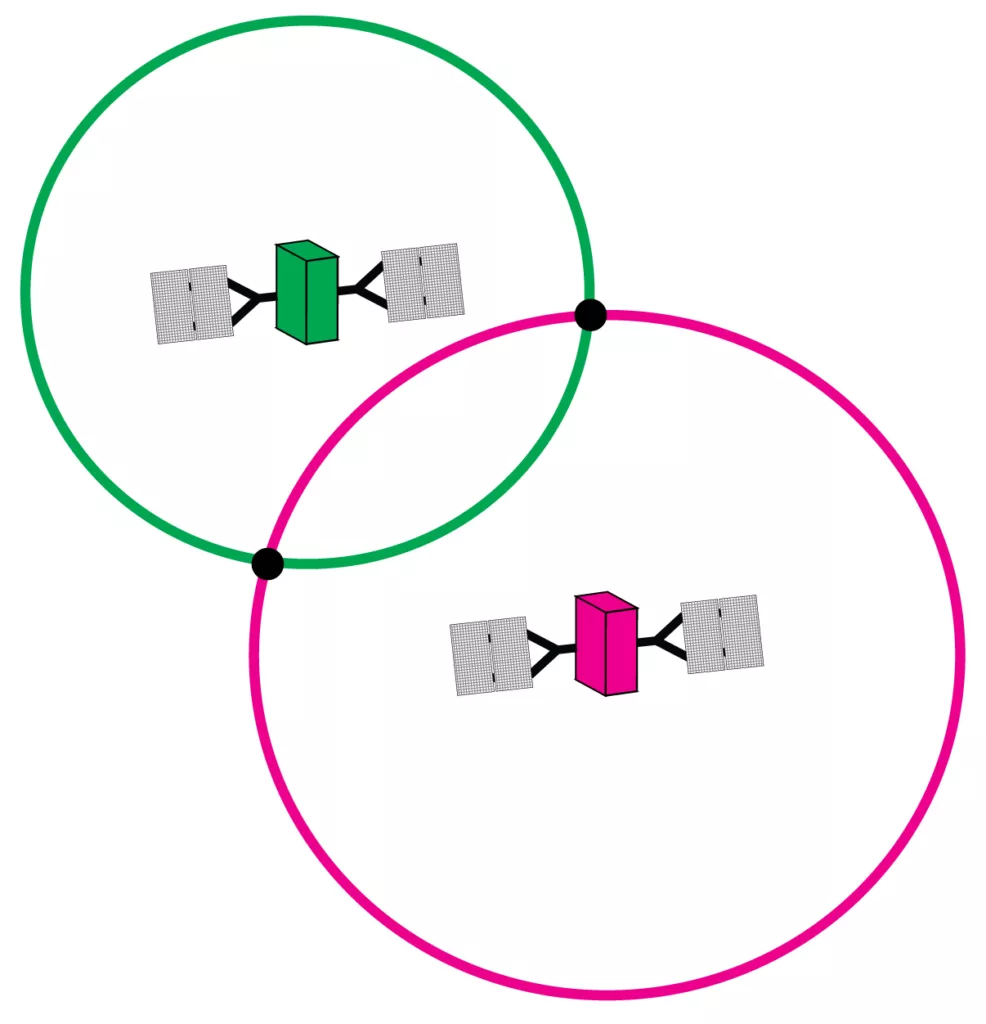
Once you do this for three satellites (assuming your receiver has an atomic clock perfectly synced with the satellites), the spheres are only going to intersect the Earth’s surface at one point—your location! With the addition of a fourth satellite, you can find your position without the need for an atomic clock in your receiver. And if you add even more satellites, the error bars on your position will shrink.
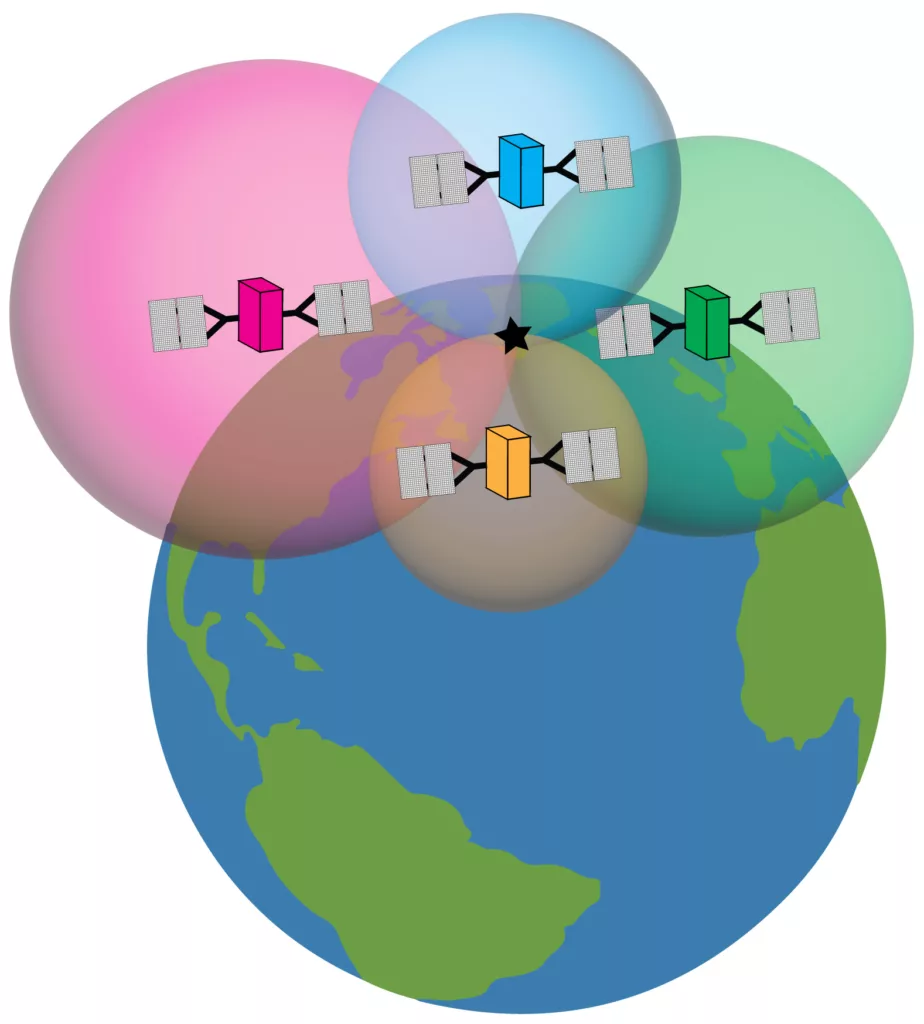
Basic GPS receivers are usually accurate to within a few meters. That’s perfectly good for most of us when traveling, but geoscientists need location information that is far more precise. For example, the San Andreas Fault moves roughly 30 millimeters per year. Geoscientists and surveyors collect an abundance of data, analyze a different aspect of the GPS signal, and apply corrections to increase precision. (See below for explanations of these techniques.)
Why go through all this trouble? Apart from the technologies enabled by high-precision positioning, there are many scientific applications. Data from the Network of the Americas—a network of permanent GPS stations from Alaska to the Caribbean—is used to study everything from earthquakes and volcanoes to weather conditions and sea level rise. By making such precise measurements, we are able to reveal critically important changes on our dynamic planet as they happen!