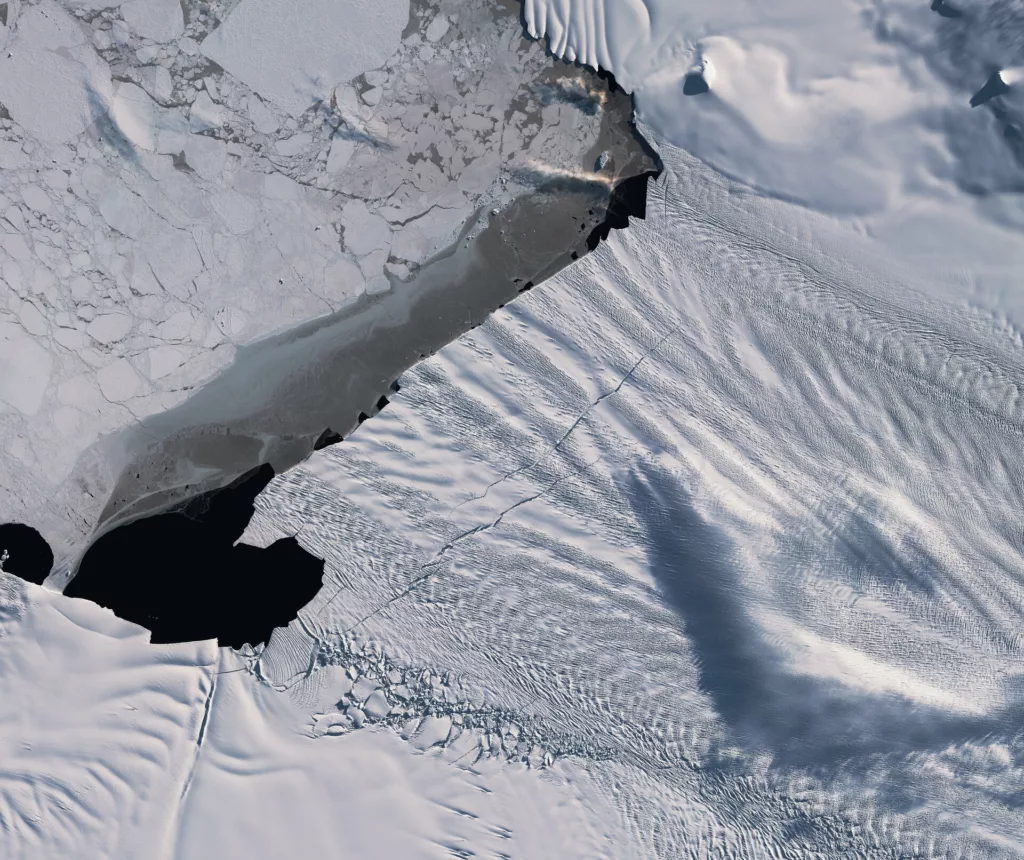
The West Antarctic Ice Sheet sprawls across much of the western half of Antarctica. The edges of ice float atop the surrounding ocean. These ice shelves buttress the ice sheet while also calving icebergs into the sea along huge fractures called rifts.
As these shelves calve and sometimes collapse, scientists most often monitor rifting via remote sensing, maintaining a bird’s eye view of the cracks that break the surface. But, direct observations of rifts during propagation remain limited. In a new paper published in AGU Advances, scientists, led by Stephanie Olinger while she was a doctoral student at Harvard University, report the first-ever seismic recording of rapid, large-scale Antarctic rift propagation.
Between remote sensing and seismic recordings, the team revealed that a more than 10 kilometer long rift in the Pine Island Glacier Ice shelf propagated at a breakneck speed of 35.1 meters per second (about 80 miles per hour). This is the fastest known ice fracture at this scale. The team also used the seismic records of elastic waves generated during propagation to estimate how fast ocean water flowed into the rift. This interaction between ocean and ice shelf may play a role in how quickly—or slowly—a rift can grow.
Fast flowing ice
Pine Island Glacier is one of Antarctica’s largest ice streams. Between 1979 and 2017, it has been the single largest Antarctic contributor to sea level rise. Since 1992, icebergs have been breaking off every two to six years.
For example, in 2015, a rift in the center of Pine Island Glacier Ice Shelf caused calving and retreat of the ice front. Put another way, the tongue of ice extending into the ocean no longer reached as far into Pine Island Bay, a nook in the Amundsen Sea, as before. Prior to the 2015 event, calving occurred in 2013 via a rift that began to break in 2011.
Watching shelves break
When a rift forms and propagates, that process typically results in the largest calved icebergs. Rift propagation has long been considered cyclical at a decadal timescale—every ten years or so—but rifting-calving cycles appear to be deteriorating.
Though understanding this cycle is important, the details of the process are murky. Remote sensing provides before-and-after comparisons, but small-scale rift behavior cannot be observed because most satellites collect images several days apart instead of hovering overhead. An image of a new rift does not tell scientists whether it propagated over several days or ripped along in minutes.
Seismic observations can help, but are limited because field campaigns can be hazardous, particularly in areas of active ice shelf deformation. Nevertheless, when seismic data is available, they provide direct evidence of what may have happened during a rifting event. Such is the case of a rift and accompanying seismic signal in 2012 on Pine Island Glacier.
Complementary datasets
Combing through satellite imagery helped the team of scientists, led by Olinger, identify a 2012 episode of rapid rift propagation for an existing crack in Pine Island Glacier Ice Shelf. With the before-and-after images, the team estimated a length increase of 10.5 kilometers and a final average width of 150 meters. Indeed, the imagery confined the rift to a 3-day time window.
Within that relatively restricted time, the team sorted through seismic data recorded by a set of stations deployed on Pine Island Glacier Ice Shelf from 2011 to 2013 (facilitated by the SAGE Facility operated by EarthScope) and found an interesting signal on 9 May 2012. The team hypothesized—and confirmed—that the rift extension most likely generated the seismic signal. This signal was recorded at seismic stations located hundreds of kilometers away, which eliminated ocean waves as a possible source. The event spectra also differed significantly from teleseismic earthquake spectra, implying that a far-off temblor could not have been the cause, said Olinger, who is now a postdoctoral fellow at Stanford. The seismic waves also abruptly ceased after 300 seconds. This “stopping phase” is highly unusual, and not observed in tectonic earthquakes.
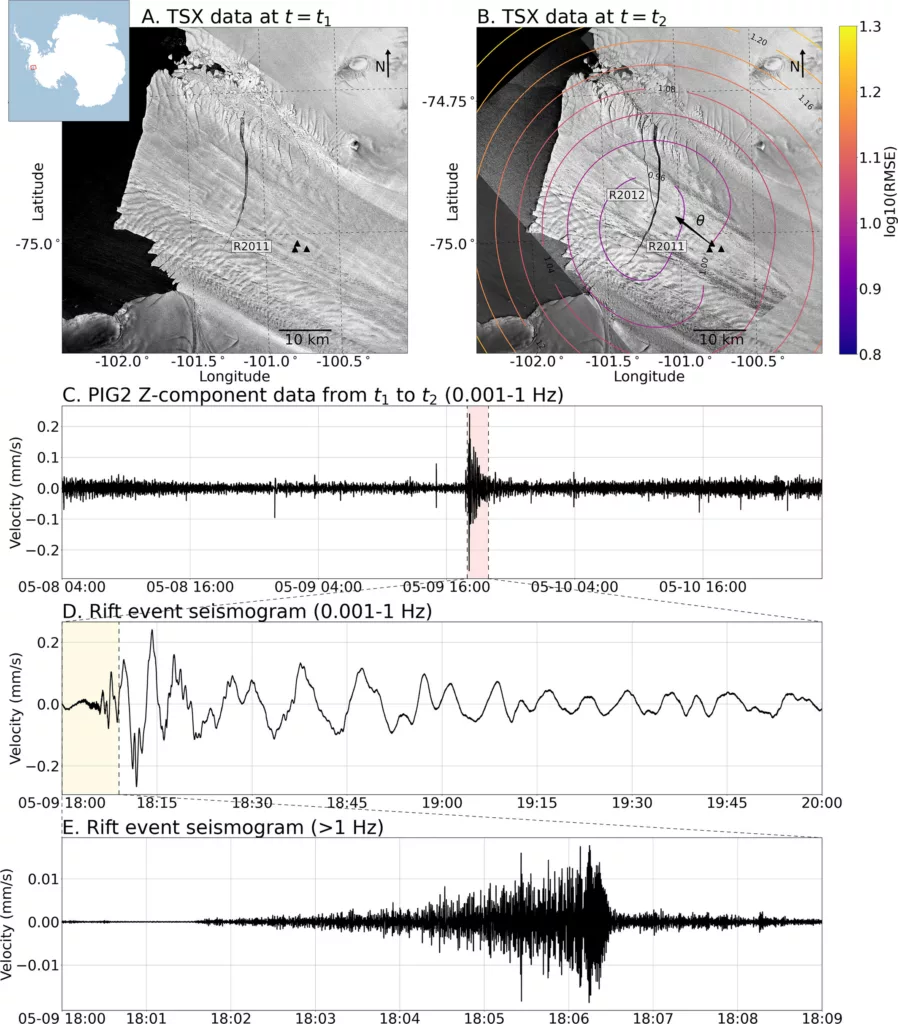
Rupture velocity
By combining the rupture length of 10.5 kilometers with the 300 second duration of the seismic event, the authors calculated the rupture velocity of 35.1 meters per second. Based on theory, ice should fracture at rates approaching 1,500 to 2,000 meters per second. Yet, the speed calculated in this study was two orders of magnitude slower.
To explore how the ocean and rift may have interacted with one another, the team used a simple model. The model equations couple brittle fracture of the ice to the flow of ocean water into the newly formed rift.
The rift is represented by an equation describing a sharp fracture in a linear elastic plate subject to uniform tension. In the model, water flows through the cavity under the shelf, into the existing crack. To initiate rifting, the team applied stress that just exceeds the fracture toughness of ice. As the rift propagates, the rift’s volume increases, with water rushing in. But the water flow isn’t fast enough to maintain the initial position of the water level within the rift. This decreases the water pressure acting to open the rift, reducing the total stress driving rift propagation. Thus, the rate of rifting slows.
With this model, the team assigned the rift an initial length and width from satellite observations, assumed a uniform ice thickness and water depth, and proceeded with calculations. The model, which is highly sensitive to the stress applied at the tip of the rift, reproduced the observed rupture velocity, supporting the hypothesis that coupling between rift and ocean limited how fast the ice could break.
“We found that, in the context of rifting, the ocean is much more than simply the foundation on which ice shelves float,” Olinger said. “We’ve known for decades that ice shelves gradually respond to ocean forcing, but our modeling shows that the ocean can exert a significant influence on processes that happen over really short timescales, like rapid rifting.”
Fast and slow
The interaction between water and ice often results in rifts, calving, and possible collapse because of the role that water plays in destabilization of fractures. However, this work demonstrates that the dynamics of water flow can also slow the speed at which a rift forms.
And yet, this study also provides the fastest observed episode of rift propagation, with the rift tearing 10 kilometers in a matter of minutes. “Is this type of rapid rifting a relatively common type of behavior that we’ve missed until now because of observational limitations, or is this a rare style of rifting that only occurs when precise conditions are met? As climate warming progresses and continues to drive changes in the Antarctic, could this type of rifting become more common?” Olinger considered. “There’s lots left to learn, but the fact that rifts can extend so quickly suggests that they could be a mechanism of rapid ice shelf change or collapse. So, it’s critical that we keep studying this process and reach a better understanding of the physics that control ice shelf rifting.”