In September of 2023, seismologists detected a strange signal. Stations around the world were picking up very-long period seismic waves, with a period of 92 seconds (this means a single cycle—from crest to trough of a wave—takes more than a minute and a half). This observation was curious enough on its own, but then things got even weirder; the signal lasted for nine days.
What culprit could possibly have caused Earth to hum so consistently for so long? As it turns out, the chain of events began with climate change and ended with water in an East Greenland fjord sloshing back and forth for a week.
A new paper published in Science, led by Kristian Svennevig of the Geological Survey of Denmark and Greenland and co-authored by another 67 scientists, explores the cascading hazards that caused the seismic signals. The authors discuss the scientific sleuthing required to rule out possibilities ranging from natural forces like undersea volcanoes to “alien slow party,” as co-author Thomas Lecocq from the Royal Observatory of Belgium jokes.
The actual cause, the team found, was a rock avalanche, induced by glacial thinning, that plunged into Dickson Fjord, triggering a 200-meter-high tsunami. That’s a little over half the height of the Empire State Building, which stands at 381 meters excluding its pinnacle. Much of the tsunami’s energy, stuck in the narrow fjord, turned into a 7-meter-high long-duration seiche. (A seiche occurs when water in sloshes back and forth. Think about someone doing a cannonball into a pool, and how—especially if the water is calm—the water splashes wildly from side to side, slowly decaying away.)
Another paper, published in August in The Seismic Record (TSR) and led by Angela Carrillo‐Ponce of the GFZ German Research Centre for Geosciences, looked at regional and global seismic signals, with the authors finding the same series of events—a rockslide that caused a megatsunami and week-long seiche in which water sloshed inside a narrow fjord.
Climate change causes more landslides
Landslides that led to tsunamis have been reported from western Greenland, and are also a concern in places like Chile, Norway, Canada and Alaska. For these regions, scientists worry about myriad climate-change feedbacks that will result in more landslides. For instance, as glaciers melt, their valleys are no longer buttressed by ice, leaving the oversteepened slopes prone to tumbling down. Plus, as permafrost proves impermanent, its degradation may also result in an uptick in gravitationally driven mass movement. Changes in precipitation could also play a role.
Regardless of the exact process driving any given landslide, when one plummets into a fjord, tsunamis pose a particular problem. The larger the landslide that lands in the water, the greater the amount of water that gets displaced—and the loftier and more destructive the potential tsunami. As these landscapes are increasingly destabilized because of climate change, scientists expect more, and possibly bigger, landslide-induced tsunamis.
Sleuthing with seismic data and satellites
On September 16, 2023, scientists observed the weird, humming seismic signal that clearly wasn’t a typical earthquake. Seismologists can use a variety of ways to determine the original seismic source in the same way they determine an earthquake’s epicenter. Both papers traced the signal’s origin to East Greenland—a place not known for landslides that produce tsunamis. At the same time, authorities in Greenland reported that a large tsunami had impacted the unoccupied Nanok station and research base at Ella Ø, which is located further down the fjord into which Dickson Fjord feeds.
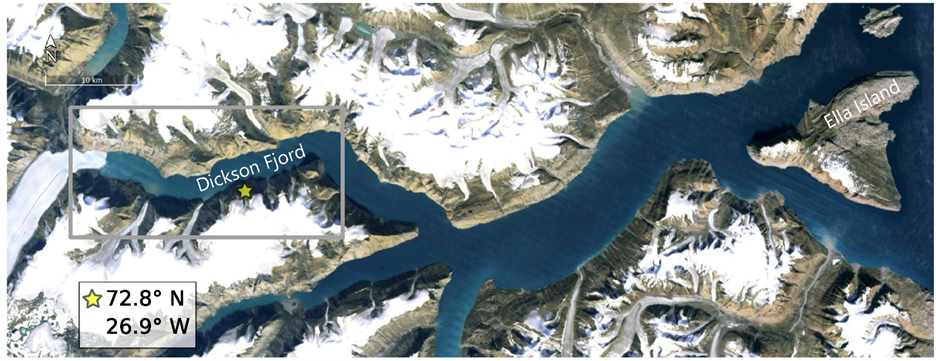
Satellite and field data from the Sciencepaper confirm that 0.025 cubic kilometers of largely metamorphic rock began its descent from about 1,200 meters above Dickson Fjord, on the southern wall. About halfway down, the rock slammed onto a 200-meter wide glacier tucked into a gully that feeds into the steep-walled fjord. The gully, bounded on either side by the towering rocks, channeled and accelerated the mix of rock and ice. When the jumble hit the water, it may have triggered a submarine landslide as well, according to the Scienceteam. They also found that the rock-ice avalanche runout distance—the distance in which a flow slows and eventually stops—was at minimum 2.2 kilometers. The team’s analysis of satellite imagery from Dickson Fjord also unearthed at least four smaller landslides, including a later one in the same gully. Each one corresponded to another monotonous (very long-period) seismic signal.
High-frequency data from nearby seismic stations contain further clues, according to the Science team—two distinct trains of seismic waves. The first signal recorded the initial rockslide. The second tracked the rock-ice avalanche moving down the glaciated gully. Both waveforms feature characteristics that are typical of landslides. The data from the three nearest seismic stations also helped the Science team estimate the trajectory of force transferred from landslide to Earth. In other words, they used seismic data to track the path of the flow from initiation on the rock wall to its travel down the gully to final deposition in the water.
Tsunami and seiche
Dickson Fjord is 540 meters deep and 2.7 kilometers wide. It begins west-northwest of the landslide’s locus, and curves from east to northeast until a sharp right turn yanks the fjord southeast. Eventually it connects with the Røhss Fjord that reaches the Atlantic Ocean.
When the mix of rock and ice reached the water, the resulting megatsunami was more than 200 meters high, according to both papers. According to the Science paper, drone data collected in the field confirm that subsequent waves to splash the glacier’s terminus were up to 110 meters high. The authors of the TSRpaper note found that the tsunami run-up averaged 60 meters along a 10-kilometer stretch of the fjord.
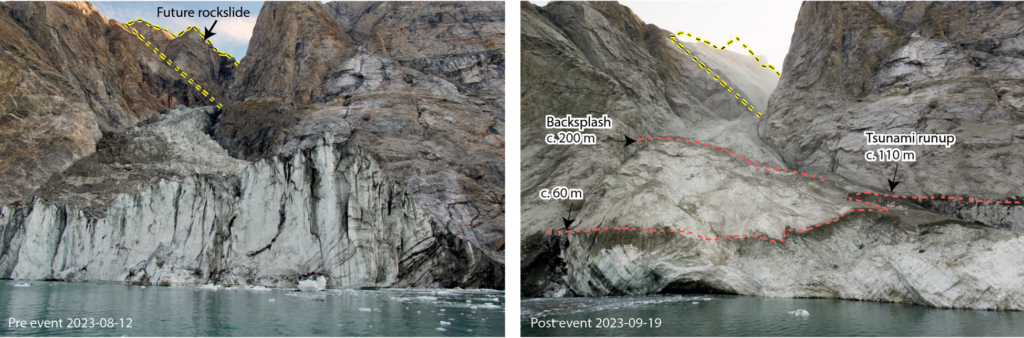
“Normally, tsunamis dissipate locally within minutes to hours,” says Stephen Hicks, a co-author of the Science paper in a video about the event. “What caused the 9-day global seismic hum?”
The Science team’s analysis of three-component seismic waveforms from stations at great distances from Greenland shows that the 9-day long signal’s pattern as it traveled around the world relates to the geometry of the fjord and the water within. For instance, snake-like Love waves dominated stations located in line with the longitudinal axis of Dickson Fjord. At seismic stations perpendicular to the fjord, rolling Rayleigh waves stood out.
This pattern, says Paula Koelemeijer, “can only be caused by a force oscillating back and forth at 90° to the long axis of Dickson Fjord.” In other words, the waves bounced from shore to shore across the short approximately 3-kilometer width of the fjord, oscillating about every 90 seconds. Stations used for this analysis include those of the Global Seismographic Network, as well as numerous other stations that are part of the NSF SAGE data archive, and other seismic networks around the world, from Costa Rica to New Zealand.
But understanding just how the tsunami became such a long-lived seiche proved tricky. Though there’s a sea level gauge in Dickson Fjord just 8 kilometers from where the landslide met the shoreline, it only samples every 15 minutes, likely missing the waves at their highest.
Numerical modeling can help. This is where scientists use lots of math and computers to simulate what happened over time. In this case, the question at hand required tsunami modeling of higher-than-typical resolution.
In the Science team’s most detailed simulation, about 5 minutes after the landslide hit the water, the fjord’s waves stabilized into a slowly decaying seiche with a slosh that matches the resonant frequency of the fjord’s geometry. The reasons it lasted for 9 days? There are a few, one of which is that the open ocean is about 200 kilometers away. But locally, the western part of the fjord ends with a glacier, and is essentially a dead end. The sharp bend to the east, along with the narrowness of the fjord’s walls meant that the tsunami’s energy couldn’t easily dissipate, Hicks says. So, the fast-moving landslide entered the fjord at about the halfway point and came in perpendicular to the long axis, launching most of its energy to the opposite wall, less than 3 kilometers away. It’s possible that the large scale of the initial wave means that the physics did not behave as we expect for lake and ocean seiches, he continues. “A sort of scale-dependency.”
Impacts
At Nanok station and the research base at Ella Ø, a runup height of 4 meters resulted in 80 meters of inland inundation. Infrastructure worth nearly $200,000 USD was destroyed. Svennevig and colleagues note that an old trapper hut—one that had never been affected by tsunamis during its century-long history—was destroyed, highlighting the unprecedented nature of this series of events.
Thankfully, though cruise ships pass near, and sometimes around, Ella Ø every week when the sea is clear of ice, none were in the fjords at the time, although they were nearby one day before and one day after the landslide. “A stroke of luck,” Hicks says.
Three weeks after this event, a similar signal originated from the same location and showed the same patterns, but with half the amplitude, according to the Scienceteam. This was yet another landslide-tsunami combination from the same gully, though smaller and less dramatic.
This event highlights the need for sea-level gauge sensors with high sampling rates to be installed in confined basins like this one. But Hicks also emphasizes the need for high quality seismic networks. “The slow seiche oscillation of about 90 seconds is right on the limit of what most seismic stations are designed to detect.” Seeing these signals requires sensors installed in quiet locations, like those of the GSN. “The Global Seismographic Network has some of the best seismic stations in the world; without them, we would not have been able to detect or characterize the highly unusual seismic signal from Greenland.”
“The analysis of the seismic signal can give us some answers regarding the processes involved and may even lead to improved monitoring of similar events in the future,” says Carrillo-Ponce. “If we had not studied this event seismically, then we would not have known about the seiche produced in the fjord system.”
Plus, says Hicks, “the high temporal resolution of seismic data (i.e., hundreds of samples per second) means that we could ultimately provide early warning of such catastrophic events.”