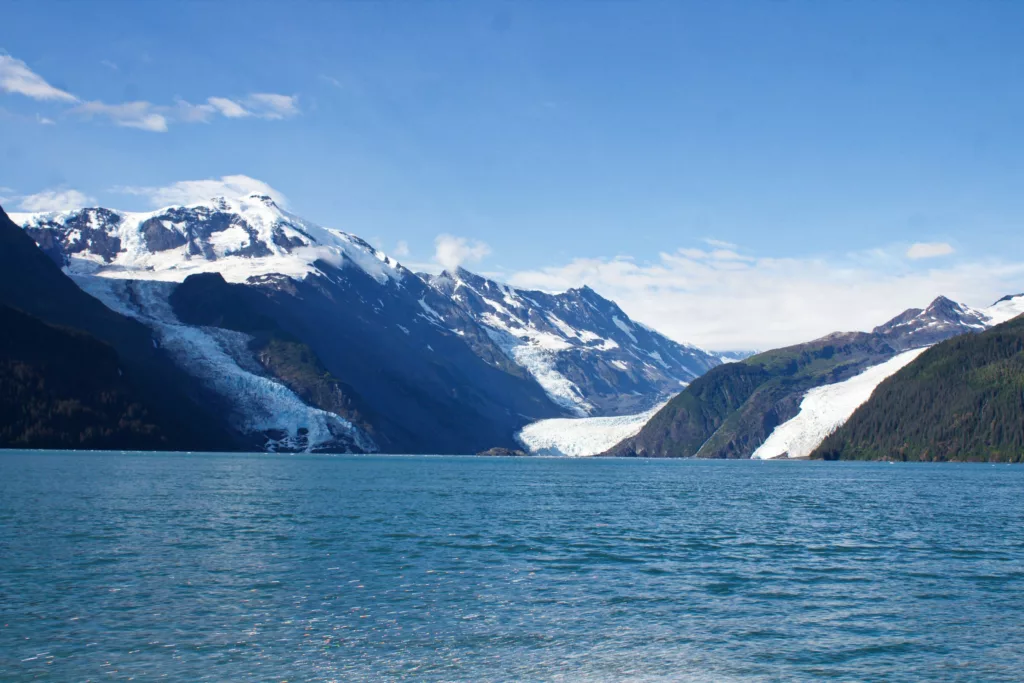
Retreating glaciers leave behind extremely steep slopes that are no longer buttressed by ice. One potential result: landslides. Worse yet? A landslide careening into the ocean, producing a tsunami.
For instance, a 2015 landslide in Taan Fiord, Alaska, sent rocks into the water, causing a tsunami that surged at least 190 meters above the waterline. A similar event occurred in Karat Fjord, Greenland in 2017, killing four people. Scientists suspect that glacial retreat will increase the number of landslides, which is of particular concern in places like coastal Alaska where steep, glaciated mountain ranges dominate the landscape.
In a new paper published in The Seismic Record, seismologists Ezgi Karasözen and Michael West, from the Alaska Earthquake Center at University of Alaska Fairbanks, explored ways to monitor these tsunamigenic landslides. The team used seismic data from nine large landslides across southern Alaska to lay the groundwork for a warning system.
Detection Difficulties
Massive volumes of rock rapidly moving downhill can create distinctive low-frequency seismic signals at stations thousands of kilometers away. Though such distant detections of seismic signals are useful for identifying landslides after they’ve occurred, the speed at which seismic waves travel render these data unhelpful for real-time detection. On the other hand, research out of Taiwan suggests that identifying, locating, and issuing alerts for large landslides could take about six minutes when using a regional seismic network.
Another complication pertains to smaller landslides, for which previous studies tended to focus on high-frequency waves. This is because low-frequency waves produced by such events are usually less distinct, Karasözen said. The high-frequency waves can be used to estimate location and volume, but relying on these signals requires knowledge of the landslide’s source, movements within the landslide body, and the ground on which it slides.
In places like coastal Alaska, additional complexities arise from competing seismic signals. Local earthquakes, crevassing or calving glaciers, and other seismic sources can all obscure seismic waves from a landslide.
“Our approach is similar to [the global approach], but we use stations less than 200 kilometers [away],” Karasözen said.
Test cases
Barry Arm, in Alaska’s Prince William Sound, poses a particular concern because of Barry Glacier’s retreat. Steep fjord walls have slumped in spurts over the past few decades, and scientists worry that a catastrophic failure might cause a major tsunami that could reach nearby communities in just 20 minutes.
To that end, Karasözen and West looked to landslides spanning southern Alaska that had both nearby seismic records and volume estimates. They used nine events as their benchmarks, including three from Barry Glacier, as well as the above-mentioned Taan Fiord landslide. This includes data from the NSF SAGE Exotic Events catalog.
The selected landslides span a range of volumes, tectonic settings, and station coverage. “We purposefully choose examples with nonideal network configurations,” wrote the authors, “because they serve as realistic edge cases.”
Seismic signals at close distances
At seismic stations located within a few hundred kilometers of the source landslide, vertical-component long-period waveforms show remarkable coherence and moveout.
“Coherence is key here, to why this approach works,” Karasözen explained. The seismic energy of a landslide looks similar at different stations. That similarity—the coherence—is a tool that can trace a landslide’s origin.
Moveout describes the difference in arrival time of a signal at stations that are different distances from the source. “Moveout is also important,” she said, because “we can see this energy at greater distances, and this allows us to use more stations to locate this source.” Short-period, high frequency waveforms are less useful, especially because they share similarities with seismic signals of nearby glacial activity and rapidly attenuate, or fade away.
Can these long period seismic waveforms help locate a landslide quickly? The team explored this question by applying three methods to near-source observations. They found that when station coverage is good, location estimates are excellent: The three methods agree with one another. Nevertheless, even with sparse station coverage, most of the other benchmark landslides were reasonably well-located, although errors for location estimates were higher.
In addition to location, a volume estimate is essential for determining if a landslide could truly produce a tsunami. This information is imperative for emergency response. The authors considered how to link seismic amplitude with landslide volume and found that larger volume landslides produced larger seismic amplitudes (after adjusting for factors like geometric spreading). Amplitude is a measurement of the energy of the signal, and seismic energy is influenced by a number of factors, including volume, height, runout, and duration.
“In a rapid response situation, volume is sufficient to assess the hazard and related tsunami potential,” Karasözen said. They had to find a way to rapidly estimate volume. To do so, they compiled amplitudes of seismic waves from the data, as well as volume estimates from published sources—retrospective studies that involved customized modeling efforts that cannot be rapidly automated. Then, they developed a linear relationship between amplitude and volume.
“Then, we looked at how good that relationship—the one that takes only amplitude into account—is at estimating the volume of each event,” Karasözen explained. In most cases, the volumes of the studied landslides estimated via the amplitude-volume relationship developed in this study compared well with volume estimates derived from more complicated scaling relationships. “But, [the relationship we derived between amplitude and volume] gives us a quick, ballpark estimate that could be quite useful in real time,” she said.
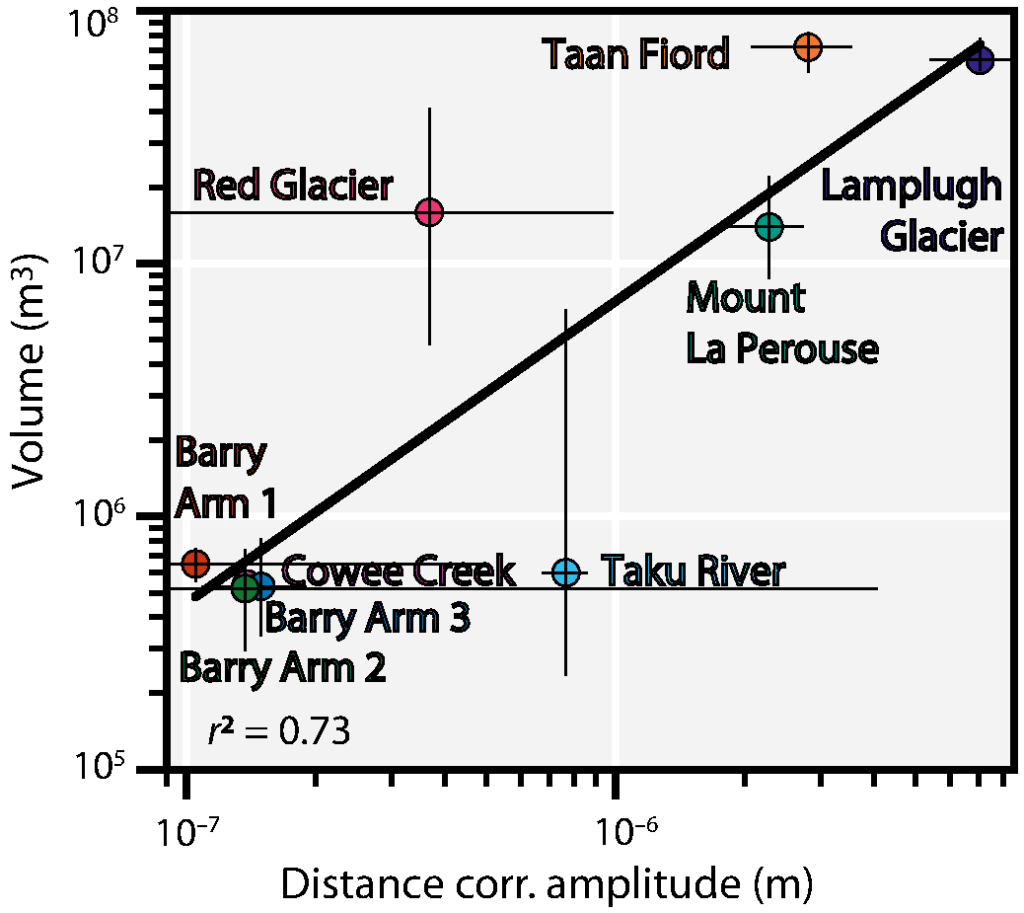
Monitoring methods
At the moment, a real-time test project has been set up on Barry Arm. Though it is not yet operational for public-alerting purposes, it forms the foundation for a future system.
Every minute, an algorithm scans for landslide signals over the prior 180 seconds of data that comes from seismic stations in and around Barry Arm. This includes stations that were originally part of the EarthScope Transportable Array. If a landslide is detected, an alert is declared (only to the authors at the moment) and the results go to the next step—the amplitude and volume routines.
During the summer of 2023, the system successfully identified seven landslides in near real-time that were confirmed by either aerial or satellite imagery. The team concluded that detecting and assessing landslide location and magnitude could be done in as little as three minutes. “This marks a promising beginning toward the systematic monitoring of landslides and potentially tsunami-triggering events along southern coastal Alaska, leveraging the existing Alaska seismic network,” Karasözen said. “While refinements are necessary to enhance accuracy and reliability, this initiative paves the way for significant advancements in landslide warning systems.”