Sometimes more precise and sensitive measurements are needed to record seismic activity and processes of deformation. Borehole strainmeters are a great tool to add to a geophysicist’s toolkit. But what are borehole strainmeters?
How it works
A borehole is a long, cylindrical hole drilled vertically into the ground. Strainmeters are traditionally used to detect “strain”, or deformation of a material, and linear strainmeters are used to measure a change in distance between two points. So, a linear strainmeter placed into a borehole can measure deformation of the surrounding rock.
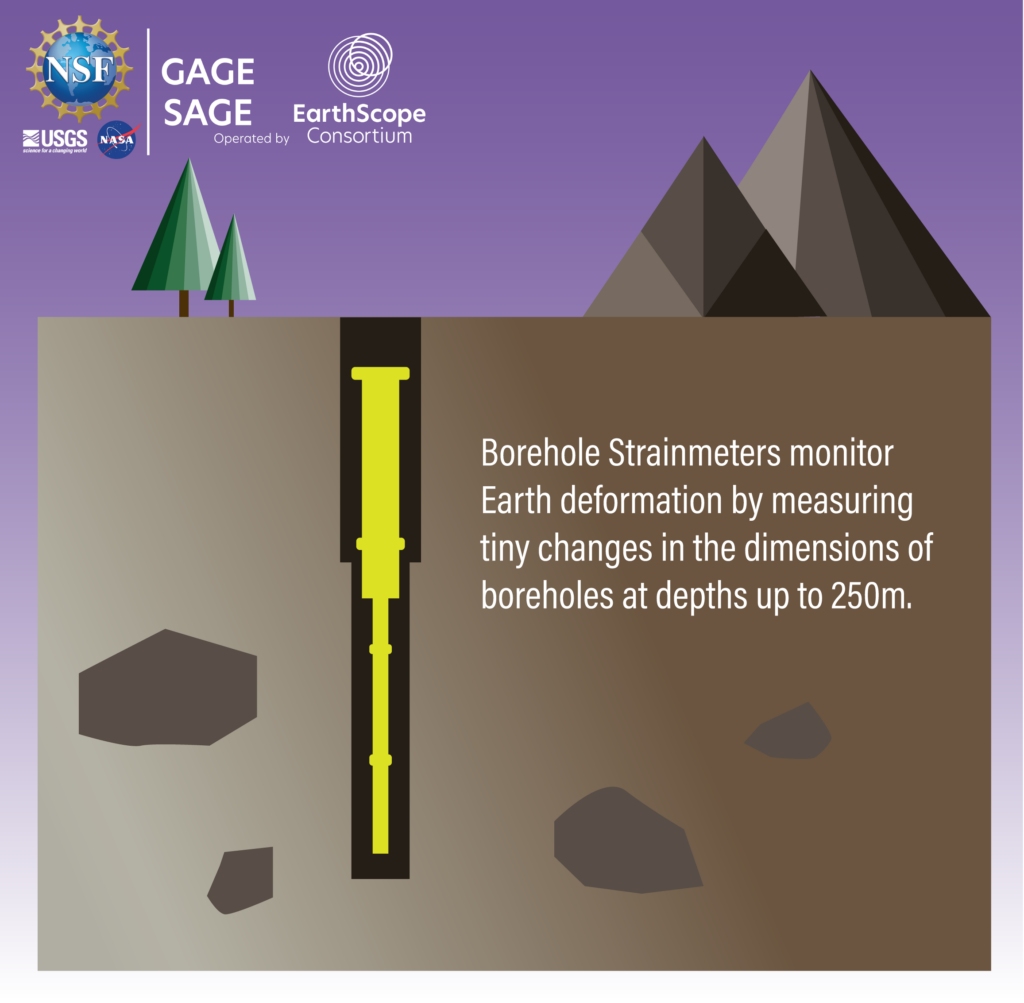
Strainmeters are capable of measuring strain changes over time periods of fractions of a second or gradual changes over years. This makes borehole strainmeters extremely useful for a variety of seismic measurements, from big earthquakes to very subtle plate tectonic motions and even tidal forces.
Borehole strainmeters have been used extensively in different research efforts since the 1980s, and comprise part of the Network of the Americas (NOTA). Within NOTA boreholes, the instruments are installed at depths of up to 250 meters below ground. NOTA boreholes can house as many as four instruments: strainmeters, seismometers, tiltmeters, and pore pressure sensors, all of which are able to collect distinct sets of measurements. These instruments can measure deformation over timescales in between the strengths seismometers and GPS units—they fill in the missing piece of information.
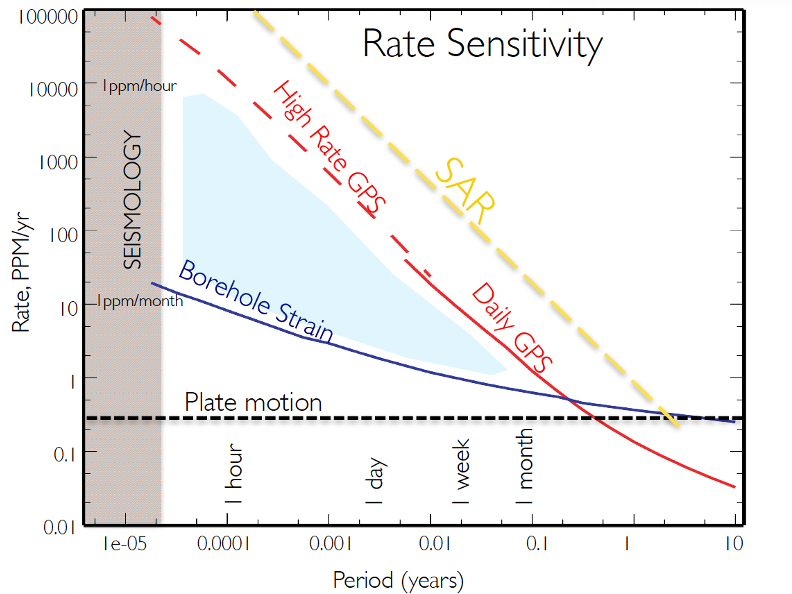
A simple yet sensitive design
EarthScope uses a Gladwin-type strainmeter in its installations. This instrument contains four gauges that measure the horizontal deformation of the Earth around the borehole in multiple directions. They register a seismic signal as an electrical signal that is then converted to a sense of linear strain on the instrument, like shortening or lengthening. It is important to make sure it doesn’t move over time, so during installation the instrument is cemented into the borehole using a special kind of expanding grout that firmly connects it to the surrounding rock. While it does a great job of that, this also means that once the instrument is installed and the grout cures, it can’t be taken out—even for repairs.
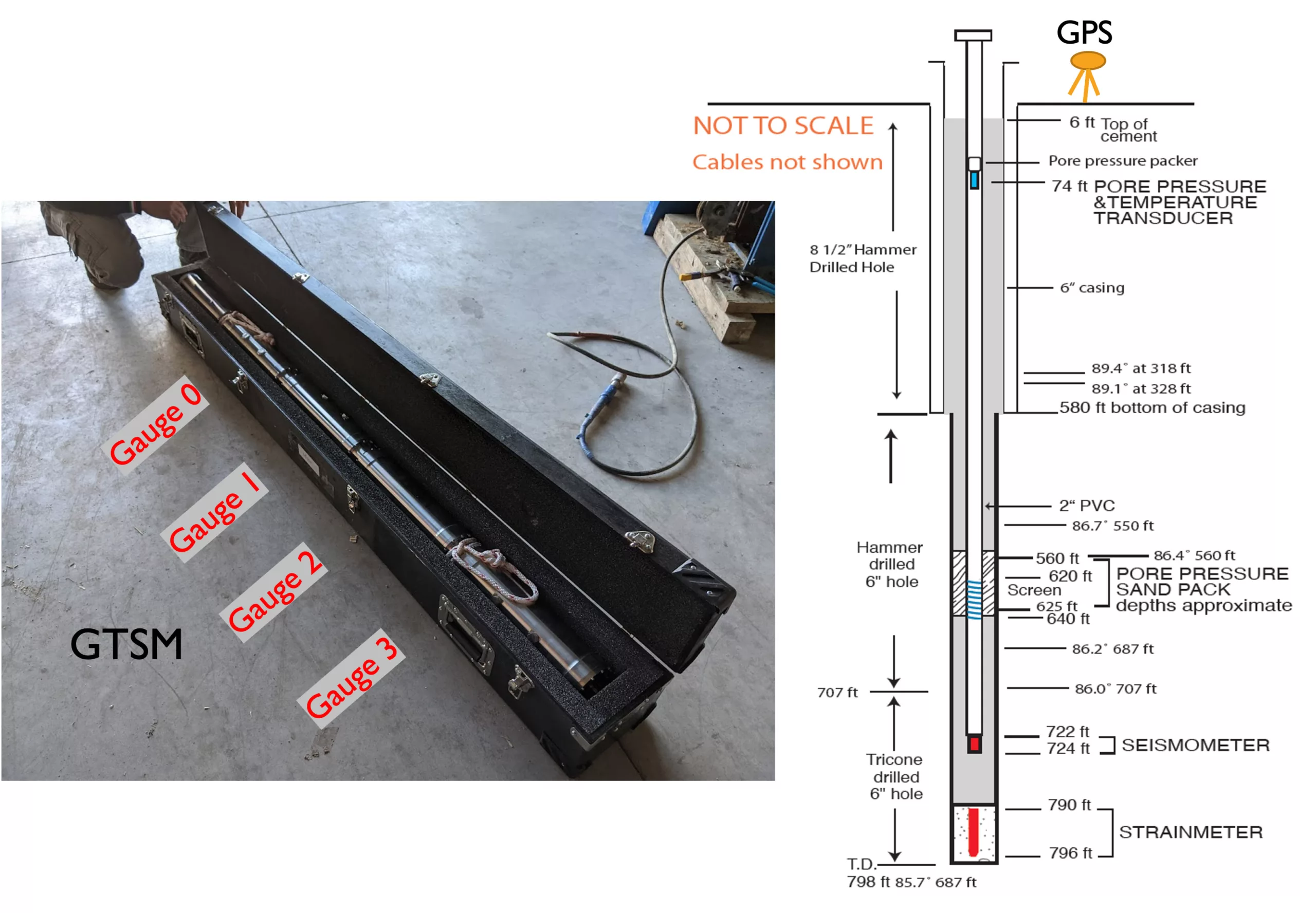
The ideal spot for a borehole strainmeter is a flat-lying area with solid rock free of fractures. If you try to install your instrument on a slope, it could be installed at an angle. If the instrument isn’t perfectly vertical, it will change the orientation of the stresses it is measuring, which will complicate usage of the data. A perfect location is also one far away from things that make noise—such as trains, the coast, or trafficked areas. Even being placed near agricultural areas can be problematic, as groundwater pumping can clutter the signal.
The sensitivity of these instruments is like detecting a 1000-kilometer-wide section of bedrock shortening by just 1 millimeter. But in order to get accurate measurements of Earth deformation around the instrument, the strainmeter must first be calibrated. Calibration of each strainmeter is typically done using Earth’s tides as a reference point. These tidal forces are the result of the gravitational pull of the Sun and Moon on the Earth. Unlike ocean tides, Earth tides take the form of a slight displacement of solid rock. By comparing the strainmeter data to a simple model of the deforming solid Earth, we can make sure the instrument is ready to accurately measure tectonic strain.
Applications
What would count as noise for a typical set of strainmeter data might actually be useful for other applications—it just depends on what the research is focusing on. For example, a pattern detected on strainmeters in Yellowstone turned out to be caused by an oscillating seiche in Yellowstone Lake likely related to hydrothermal activity beneath the lake. This has been used to study the physical properties of the bedrock in Yellowstone, and can help monitor changes in the hydrothermal system.
For another example, the TABOO-STAR strainmeter array in Italy—installed to study seismic hazards—seems to have also measured a signal related to groundwater pumping in the area. Some CO2 degassing in the mountain range has also been detected, possibly due to a subducting carbonate platform, through borehole strainmeter measurements.
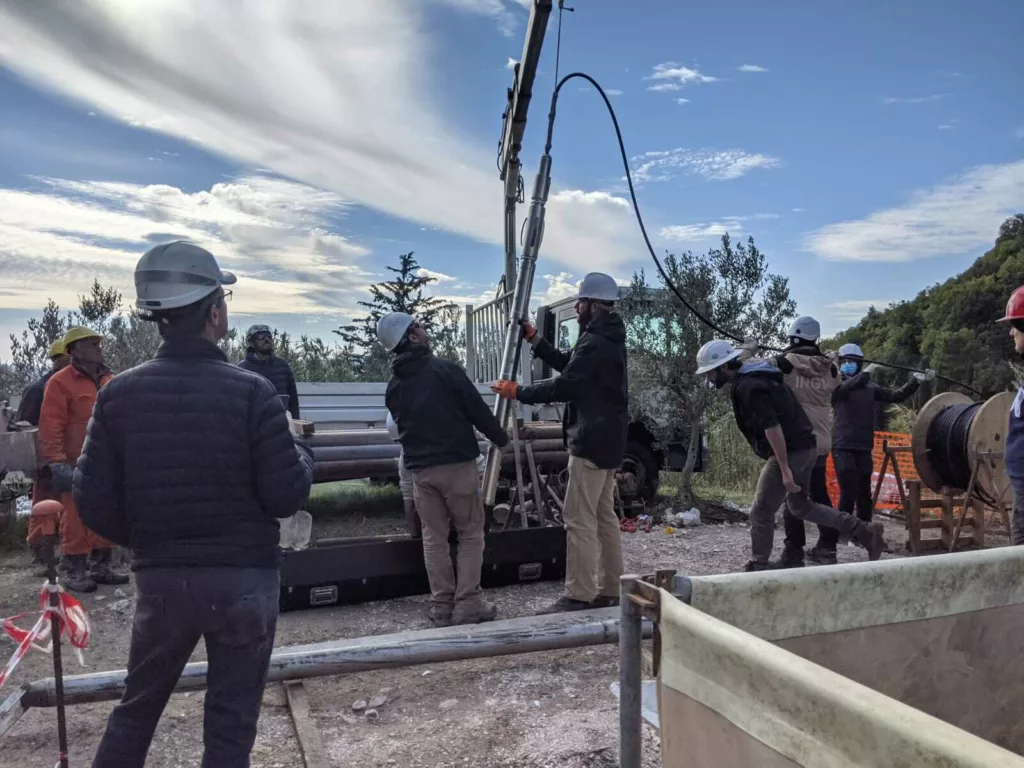
More conventionally, strainmeters installed near the Central San Andreas Fault in Parkfield, California are measuring subtle fault processes. By studying how different sections of the fault behave between and during earthquakes, we can better understand the local seismic hazard.
The unique characteristics of strainmeters complement other instruments like GPS instruments and seismometers, enabling them to help answer scientific questions by giving us a more complete picture of Earth processes.
Go deeper
To find strainmeter data and other technical resources, visit our Borehole Instruments page.