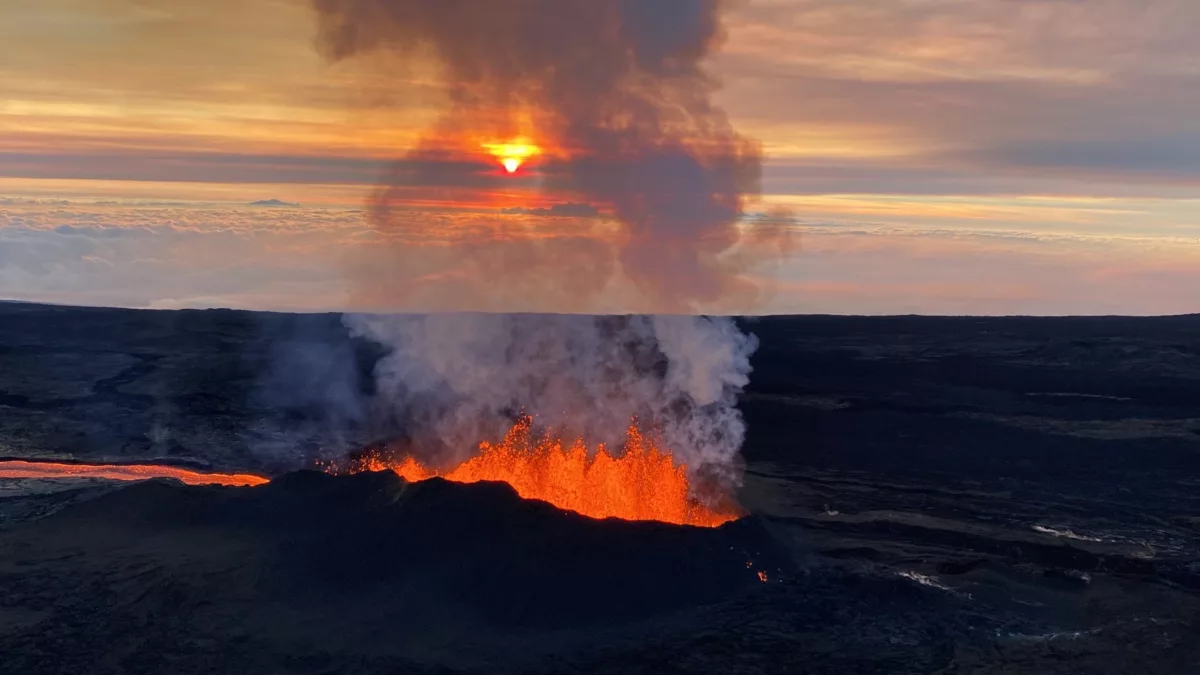
Sometimes volcanoes briefly rumble before resting again. Other times, that grumbling presages a full-blown eruption.
Distinguishing between intermittent unrest and an eruption’s prologue poses a major challenge to volcanologists responsible for forecasting earthquake activity. A recent eruption of Mauna Loa, Hawai’i—Earth’s largest active volcano—has provided scientists a unique opportunity to tackle this problem. The well-instrumented volcano began erupting on November 27, 2022 after decades of on-and-off rest.
In a new paper published in Nature Communications, a team of scientists, led by Kendra Lynn, a volcanologist and petrologist at the Hawaiian Volcano Observatory (HVO) of the U.S. Geological Survey, presents a comprehensive study of several datasets that, together, track how precursory activity at Mauna Loa proceeded prior to eruption.
Specifically, they collected information from lava, minerals and gas that tracked what was happening inside the volcano before and during the eruption. They correlated the geochemistry with real-time monitoring datasets to understand what’s in the volcano’s plumbing system and how fast it moved around. The result: a cohesive picture of the two months leading up to the eruption, as well as indicators that might be useful for rapidly differentiating between unrest and imminent eruption.
Forecasting at massive Mauna Loa
Real-time monitoring of volcanoes typically involves seismic, geodetic, and gas measurements that help scientists infer where magma is stored and where it’s going. But unrest—upticks in earthquakes and ground deformation, as well as occasional outgassing—tends to wax and wane as magma moves underground in sometimes sudden spurts.
Volcanologists have a tricky task of heralding impending eruptions that could be days, weeks, or even months away. Differentiating between general unrest and eruption run-up is a particular problem at frequently active volcanoes like Cumbre Viejo in the Canary Islands and Nyiragongo in the Democratic Republic of Congo.
Mauna Loa—one of six volcanoes on the Big Island of Hawai’i—is the second highest peak on the island, at 13,680 feet. The summit of the volcano, dubbed the Moku‘āweoweo caldera, served as the source for the last 34 recorded eruptions.
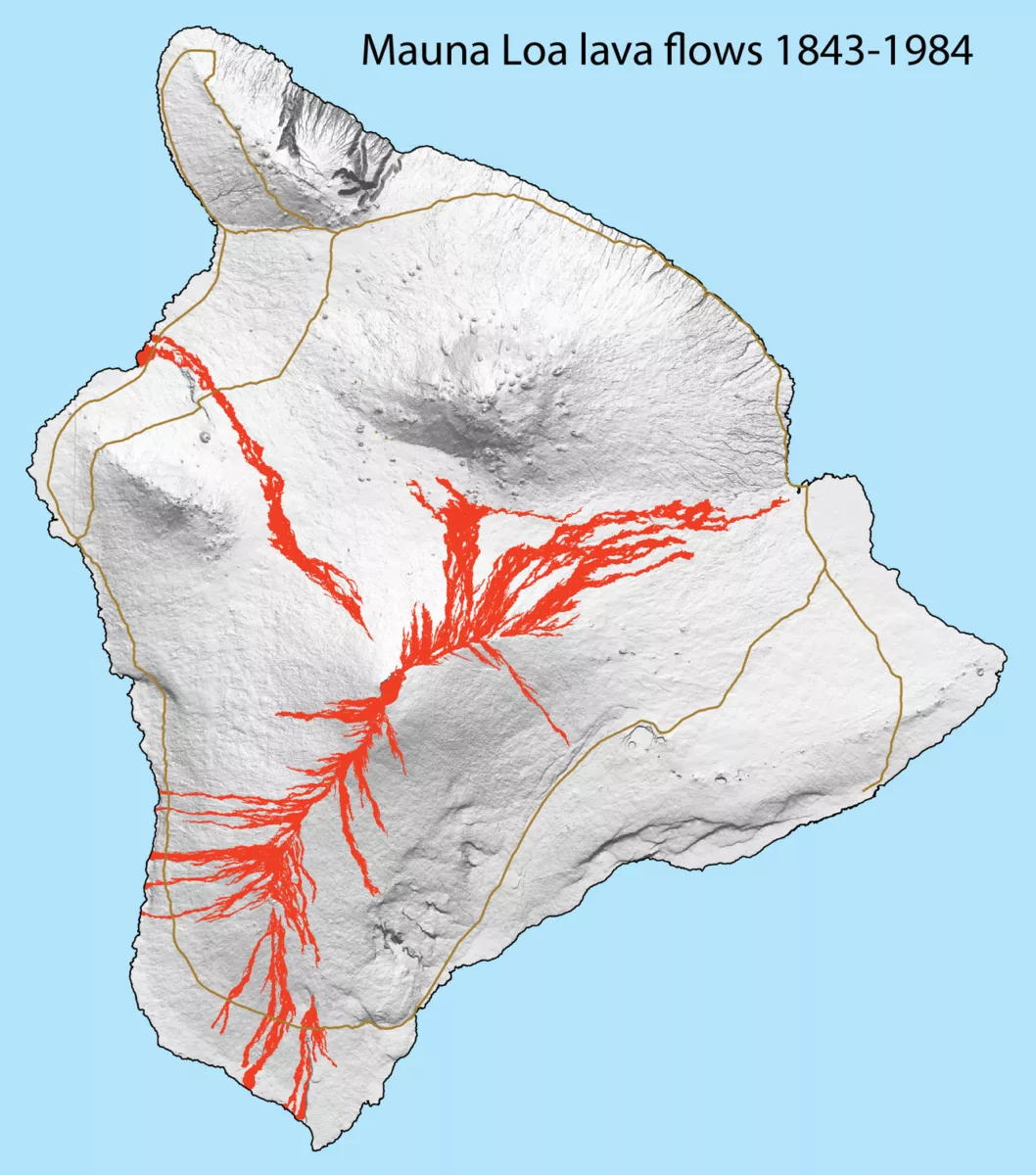
Mauna Loa’s lava is often voluminous and of low viscosity—it moves quickly and there’s a lot of it—which means communities residing on the volcano’s flanks must be vigilant during times of unrest. “It makes up about 50% of the island of Hawai’i,” Lynn said, with the major city of Hilo, among other populated areas, built atop its flows. Understanding how—and how fast—magma travels from its mantle source to below the summit caldera, can help scientists and the public alike prepare for eruptions.
The HVO keeps a close eye on all Hawaiian volcanoes, communicating volcano alert levels with the local population and air traffic control. Because periods of unrest occur more often than actual eruptions, the alert level at Mauna Loa moves between “normal” and “advisory,” (these correspond to green and yellow on the Aviation Color Code) and has done so several times between 2002 and 2022. But it’s difficult to know whether any particular pulse would lead to eruption.
The 2022 eruption
On the evening of November 27, 2022, about 30 minutes before the eruption began, seismic sensors detected a phenomenon called tremor, which tells scientists that fluid is on the move. When tremor starts, explained Lynn, “it’s often an indication that magma is moving within the volcano, potentially toward the surface to initiate an eruption.” When lava reached the surface and erupted through fissures, the eruption officially began.
The first fissures rent the southwest corner of Moku’āweoweo, the summit caldera. They propagated to the northeast, crossing the caldera, and then extended the other way toward the Southwest Rift Zone. These fissures were active for about 6 hours before summit activity waned. A dike propagated into the Northeast Rift Zone in the morning hours of November 28. By December 2, a single active vent—dubbed “Fissure 3”—became the focus of the eruption, producing lava fountains and flows. By December 8, eruption intensity decreased. On December 10, after 13 days, the eruption ended.
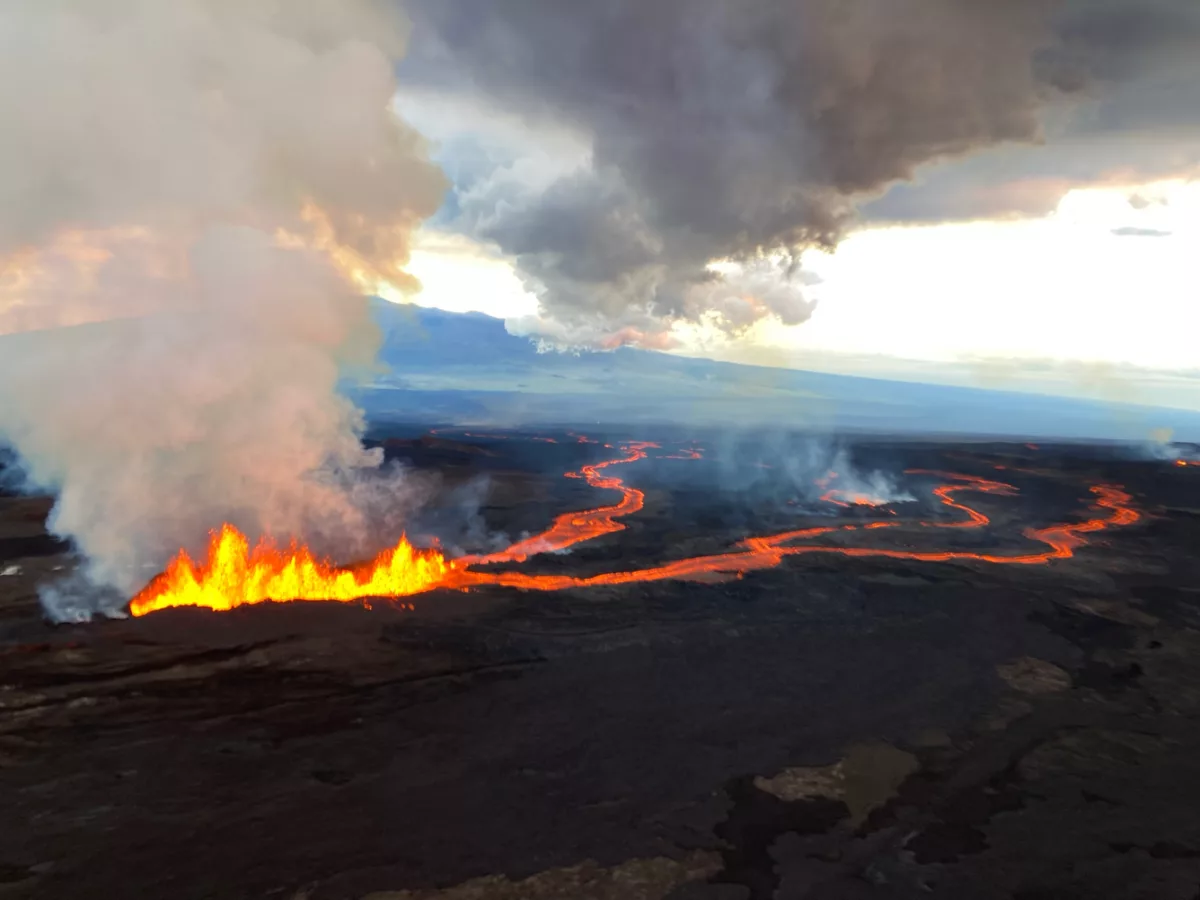
Datasets galore
Mauna Loa’s most recent previous eruption occurred in 1984, before the installation of the modern monitoring network. The 2022 eruption is the first for which multiple types of state-of-the-art sensors collected information both before and during the event.
Monitoring datasets include eruptive plume gas measurements, earthquake locations, seismic velocity changes, and ground tilt and deformation. (The seismic and GPS data are available in the NSF SAGE and GAGE data archives.)
But HVO also collected physical samples during and after the eruption. Magma is a mix of molten rock and mineral crystals, and those minerals may have formed in various parts of the volcano’s plumbing system or been plucked from the surrounding rock. Upon eruption, magma turns to lava, which contains mineral crystals that can preserve hints of their histories. HVO collected samples from flows. Chemical analysis of lava samples indicated that they were largely the same, with less magnesium (geochemists say that this is “more evolved”) than any eruption in the past 200 years. The team interpreted this as indicating magma storage in a long-lived, steady-state reservoir—rather than fresh, newly arrived magma—at a depth of about 3 kilometers.
Lava not only flows, but drops (and sometimes chunks!) are also spewed into the air, where they quench quickly, forming particles of glass that tells the scientists what the magma was like at that time. HVO sampled the material that rained from the sky both during and after the eruption. “When possible, we deploy a system of buckets to catch ash as it falls, so that we have a time series of the eruption,” Lynn said.
The scientists crushed these ash samples up and separated individual mineral crystals that had been erupted within the lava. They found extremely rare olivine and enstatite (a kind of magnesium-rich pyroxene) crystals that were large enough to measure zoning patterns of the elements iron and magnesium.
Varying states of happiness
Examining crystals’ cores and rims for changes in iron and magnesium helps determine if the crystals were in equilibrium—if they were “happy” in the most recent magma around them. The erupted glass serves as a proxy for that magma, said Lynn.
Using olivine as an example, coauthor Penny Wieser, an igneous petrologist and volcanologist at the University of California, Berkeley explained the measurements from the glass help them calculate the “happy” olivine composition. “We then compare the natural olivine to that theoretical happy composition.” If calculation and measured olivine composition match, this signals equilibrium.
“When crystals enter magmas of different compositions, they are out of equilibrium,” Lynn said, and “are ‘unhappy.’” Given enough time, they’ll equilibrate, with the enclosing magma matching mineral rims, and maybe even mineral rims matching their cores.
Lynn and colleagues found that no part of the olivine crystals were in equilibrium with the erupted glass. “The olivine crystals were the only material that clearly recorded the deeper, hotter, magma history,” Lynn said.
For the enstatite, curiously, only the core matched the glass—that proxy for the magma. This is called reverse zonation, where only the insides of the enstatite were “happy” or in equilibrium.
Taken together, these data suggest that the enstatite did not come along with the olivine crystals. Instead, the enstatite may have belonged to shallow magma stored prior to the olivine-bearing intrusion. That intrusion changed the magma chemistry, causing the enstatite to become reversely zoned, Lynn explained.
Because the chemistry of the crystals changes via a process called diffusion, which occurs at known rates at different temperatures, the team could convert the chemical differences into time. A crystal’s clock stops when it is expelled from the volcano because that process of trying to reach happiness ceases; temperatures at Earth’s surface are too slow for measurable diffusion.
Of the calculations for the time at which interaction commenced between the crystals and chemically distinct magma, 81% pointed to less than 10 weeks. This indicates that the crystals entered the system in mid-to-late September, 2022.
Fluid inclusion analyses hinted at the depth at which the enclosing crystals grew, suggesting two separate depths for magma storage. Gas measurements of the eruptive plume support the existence of separate upper and lower magma reservoirs.
Tipped toward eruption
Geochemical analysis of erupted samples can, perhaps obviously, only be carried out after an eruption. But these analyses, the authors note, “can improve scientists’ ability to forecast future eruptions by providing otherwise unobtainable insights into pre-eruptive magma storage and transport.” In other words, the only way to understand the inner machinations of eruptions is to study them in detail after the fact and apply what’s learned to future eruptions.
Indeed, this sequence of events and magma depths correlated with geophysical trends. For instance, seismic velocities decreased 10 weeks prior to eruption while seismicity rates reached their peak. The combination of instrumental and geochemical data suggest a 2-month-long sustained magma intrusion that ultimately triggered the eruption.
Several periods of unrest have occurred at Manua Loa since the 1984 eruption. But what changed to tip 2022’s magma movement to eruption?
The trigger, the authors propose, was migration of enough magma into the upper reservoir to exceed the point of failure. Simultaneous changes in earthquake frequency, earthquake location, slowing seismic velocities, tilt signals, and increased GPS-measured inflation rate all indicated magma pushing to shallower levels of the system. The key monitoring signal of shallow subsurface magma seems to be a combination of changing tilt and slowing seismic velocities.
“Future eruptions might be preceded by tilt meter and GPS signals with contemporaneous summit and flank earthquake swarms,” the authors wrote. These signals tell scientists magma isn’t far from the surface, and that eruption may be near.