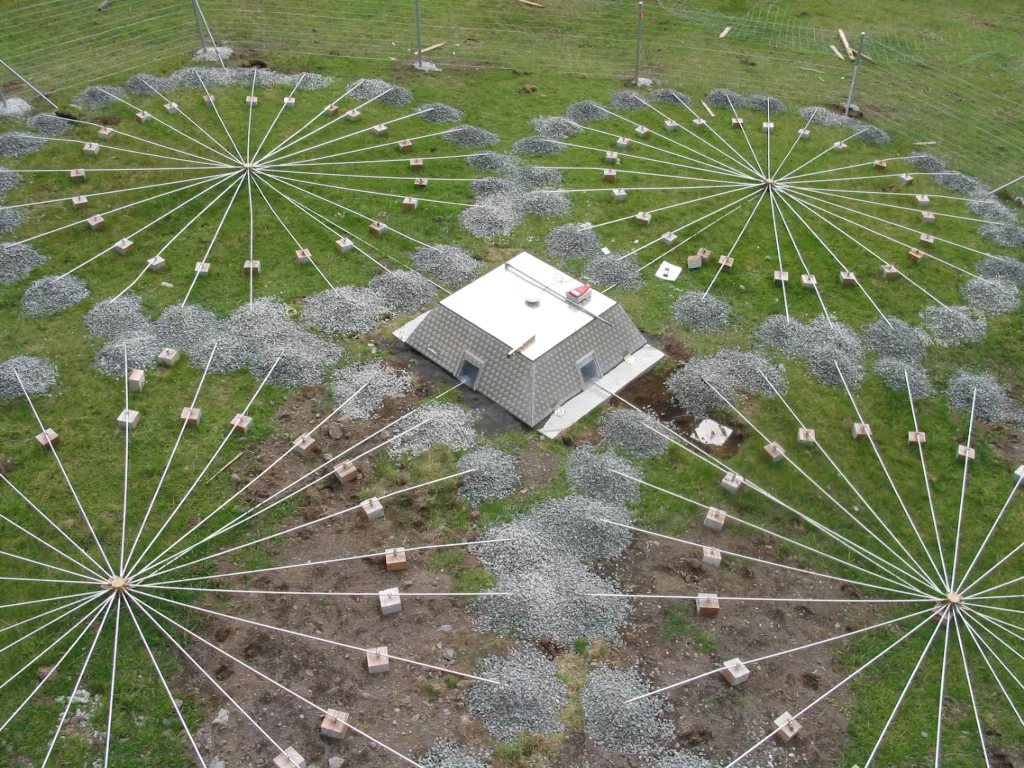
After the detonation of the first nuclear test in 1945, the world entered an unprecedented period. As countries raced to develop nuclear weapons, monitoring the detonations of nuclear tests became paramount. Over time, these efforts have grown more sophisticated.
The Comprehensive Nuclear-Test-Ban Treaty (CTBT), which prohibits all nuclear explosions in any environment regardless of yield, has been signed by 186 countries, but has not yet entered into force. In order to monitor compliance with the treaty, the CTBT Organization’s Preparatory Commission is developing ways to verify nuclear explosions. These include the International Monitoring System, which collects data, and the International Data Center that’s responsible for sharing this information.
The International Monitoring System uses four complementary monitoring technologies— seismic, infrasound, hydroacoustic, and radionuclide. Seismic stations around the globe can detect ground motions unique to nuclear explosions. Infrasound sensors listen for acoustic waves traveling through the atmosphere. Hydroacoustic monitoring measures underwater explosions. Radionuclide sensors measure distinctive radioactive material, both particulate and noble gases, that may be present in the atmosphere after nuclear explosions.
Few comprehensive datasets exist in which multiple observational methods detect well-characterized explosions and other events that would be present in any real monitoring scenario. Such benchmark datasets are needed to test advanced processing software—algorithms that can rapidly detect explosions and alert the appropriate authorities. In a paper published in Bulletin of the Seismological Society of America, Rebecca Rodd, a geophysicist at Lawrence Livermore National Laboratory, and colleagues present such a dataset, which is available via the SAGE data archive.
DAG-4 in the desert
Detecting explosions of about 1 kiloton or greater from a distance anywhere in the world using the usual suite of sensors—seismic, infrasound, hydroacoustic, or radionuclide—is well established. Smaller explosions prove more problematic to parse and must be detected by nearby sensors.
In order to support nonproliferation efforts, scientists at various U.S. National Laboratories seek to develop algorithms to detect such explosions. But to do so, they needed an appropriate dataset to test them with.
“A benchmark dataset must include observations from multiple sensing [methods] that recorded at least a single ‘known’ explosion,” explained Rodd. A well-characterized, known explosion provides a baseline against which to compare algorithm results. “This is known as ground-truth,” she said. The DAG-4 chemical explosion was an excellent candidate.
DAG stands for Dry Alluvium Geology. DAG-4 was part of the Source Physics Experiment, and was the fourth monitoring explosion at the Nevada National Security Sites. For the test, scientists collected data from accelerometers, geophones, seismometers, infrasound sensors, magnetometers, and distributed acoustic sensors deployed nearby for two weeks. This timeframe included the ground-truth DAG-4 explosion, as well as a variety of background “nuisance events” sensed both before and after. These nuisance events included background seismicity, other explosions and events related to mining activity, and “mine-like” events without a known source, Rodd explained.
The DAG-4 shot was the second largest of the four DAG explosions, with a 10-ton chemical source buried 51 meters below the ground—comparatively shallow to other experiments. The explosion, which occurred on June 22, 2019, produced infrasound signals and an air shock. The chemical explosion byproducts generated an electromagnetic signal that traveled at the speed of light. And of course, the explosion produced seismic waves in the ground. Such records were collected from a mere 10 meters away to distances greater than 250 kilometers.
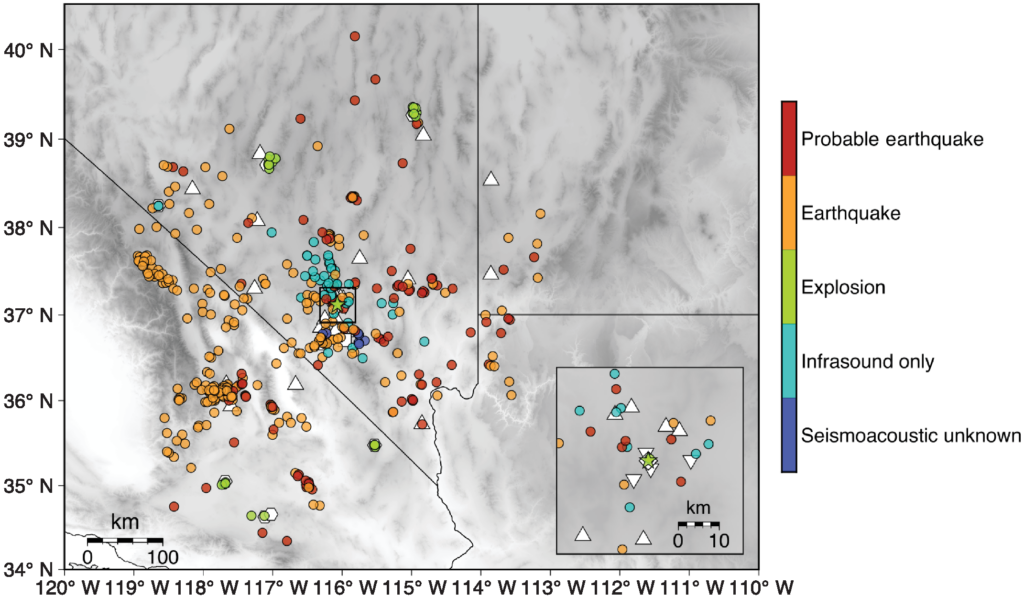
Developing dataset
The team focused on a subset of the data collected in the DAG-4 experiment—seismic, infrasound, and magnetometer information only. Other available data, such as near-source seismic sensors, were excluded because the purpose was to replicate a monitoring scenario for development of signal processing methods.
To that end, an expert analyst manually built a catalog of events that are presented in this paper. In total, the catalog includes 485 seismic, seismoacoustic, and infrasound-only events extracted from 29 seismic and infrasound sensors.
The analyst differentiated between earthquake and explosion sources without the use of software designed to discriminate between the two. Instead, if an event had multiple characteristics of an explosion and was located at a known, usually shallow explosion location that occurred during the day, the event was identified as an explosion. Events that did not fulfill the explosion criteria but were not earthquakes included in the ANSS catalog were left unassigned, but are likely earthquakes, according to the team.
Of the 485 events, 295 were earthquakes, 104 were probable earthquakes, 25 were explosions, 54 produced infrasound sans seismic waves (which the authors suspect were related to activities at the Hawthorne Army Ammunition Plant), and 7 had both infrasound and seismic signals but were associated with some other unknown source in the area.
The catalog released via this publication includes waveforms from those seismic and infrasound sensors, as well as data from two magnetometer stations. The electromagnetic signal detected by the magnetometers in particular may be useful because that signal travels at the speed of light—much faster than acoustic, speed-of-sound waves. Those data may provide a better estimate of source origin time than the other datasets, the authors say. “We hope that researchers within the explosion monitoring community can use this dataset in their development of monitoring algorithms that focus on improving detection of smaller events that could otherwise be undetected,” said Rodd. “Additionally, we saw this as an opportunity to show the value in publishing stand-alone articles on dataset releases.” Hopefully, the community will answer the call as they work to create a new generation of data processing algorithms that can detect explosions and help keep the world safe.