Subduction zones play a crucial role in recycling old oceanic crust, while also carrying water into Earth’s interior. With seismic data, scientists can indirectly observe fluid distribution beneath our feet. However, magnetotelluric imaging of electrical resistivity—which measures how strongly a material resists electric current—can help scientists better see where fluids have accumulated in the crust.
In a paper published in Nature Geoscience, researchers led by Gary Egbert, a geophysicist at Oregon State University, explored the distribution and connectivity of fluids in the central Cascadia subduction zone using magnetotelluric data collected from nearly 400 sites, including 64 offshore sensors. The team found that the rock type of the overriding crust can play a critical role in controlling fluid movement and storage. Moreover, they demonstrated that magnetotelluric data could help map out those subsurface rock types, which are key to understanding the behavior of rocks in subduction zones—the source of some of the greatest earthquakes.
Trench to arc
From an aerial perspective, a subduction zone margin often follows a pattern: trench, accretionary complex, forearc, and volcanic arc. The trench is a chasm that marks the interface where one tectonic plate dives below another. Next to the trench typically resides an accretionary complex—a zone of crumpled sediment and rocks that have been scraped off the down-going slab and become somewhat stuck in the subduction zone. Feeding sediment into the accretionary complex are rivers that thread through the forearc basin. The source of the sediment those rivers carry is usually the high-standing mountains of a volcanic arc.
The Cascadia Subduction Zone lies off the coast of northern California, Oregon, Washington, and southern British Columbia. Here, a very hot, young oceanic plate slides beneath North America. The trench and volcanic arc receive a lot of attention because of their respective earthquake and volcanic hazards. However, the boundary between the plates is a three-dimensional structure, and the megathrust—capable of producing earthquakes of magnitude 9—extends below the accretionary complex and forearc.
Cascadia’s central forearc is also distinctive because tens of millions of years ago, a thick block of oceanic crust became stuck to the western margin of North America. Called Siletzia, this area displays curiously low levels of crustal seismicity.
Data trove
To explore the fluids underfoot, Egbert’s research team accessed magnetotelluric data via the SAGE Facility operated by EarthScope. These data include information from 144 MT-Transportable Array sites, legacy profiles, and a new array deployed both onshore and offshore, said Egbert. The data extend from the oceanic spreading ridge in the west to the backarc (the region behind, or east of, the volcanic arc) in the east, and from northern California in the south to southern British Columbia in the north.
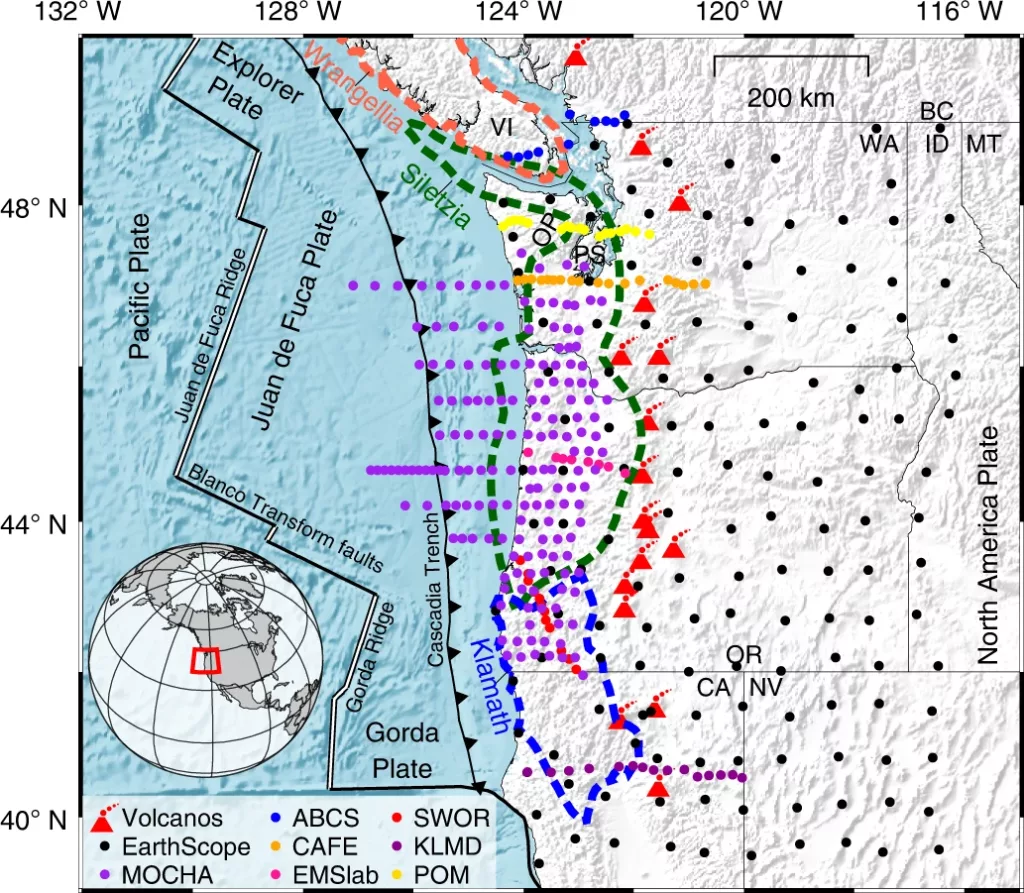
Magnetotelluric sensors can find water because rocks resist electric current flow (high resistivity, low conductivity), whereas saline water replete with dissolved ions conducts current (low resistivity). “Water has to have dissolved ions to be electrically conductive, but this is what would be expected for hot water in contact with rock for millions of years,” Egbert said. By combining indications of accumulating fluid in the data with other information, fluid transport from the subducting plate to the overriding crust can be inferred, he explained.
Egbert and colleagues focused on the forearc in Oregon and southwestern Washington where data density is highest, with a goal of looking for three-dimensional variations in large-scale, deep crustal resistivity. Where is the water, and what are the rocks?
Stuck under Siletzia
Beneath coastal Washington and northern Oregon, Egbert and colleagues found a conductive region that extends about 50 to 70 kilometers offshore. The team interpreted the conductive region as water-logged sedimentary and metasedimentary rocks of the accretionary complex that’s being thrusted and metamorphosed below North America.
In 3-D, the conductive region extends under the continent and abruptly terminates at about 20 kilometers depth. The sudden stop coincides with a massive, resistive region whose characteristics are consistent with mafic rocks of the Siletzia terrane.
Egbert and colleagues also found that resistive signals attributed to Siletzia extend to the plate interface beneath North America in patches rather than as a single continuous feature—consistent with Siletzia’s possible origin as either an oceanic plateau or a chain of seamounts. Each resistive region could represent the roots of a former volcanic center.
The distinct difference in resistivity—and therefore fluid storage capacity—between Siletzia and the accretionary complex suggests that magnetotelluric measurements can effectively map different rock types. This helps scientists constrain spatial variations in the mechanical properties of the crust, which is critical for improving our understanding of the region’s seismic hazard, Egbert said.
Water on the move
The highest conductivities imaged in this study imply a high concentration of fluids in a narrow band just updip of where highly resistive Siletzia extends to near the top of the subducting plate. In other words, as fluids are released at great depths, instead of traveling upward through Siletzia, they travel along the subduction channel before diffusing into the sedimentary and metasedimentary rocks of the accretionary complex. Egbert and colleagues thus infer that Siletzia is impermeable. The depth at which fluids are released beneath Siletzia—about 40 kilometers—is also where a special kind of slip called “episodic tremor and slip”. At such depths, rocks usually flow instead of break. However, these peculiar tremors likely represent a return to brittle deformation, almost certainly resulting from high fluid pressures counteracting some of the crushing stress.
“The area where Siletizia is has significantly less episodic tremor and slip,” said Egbert, “so if fluids can’t escape upward through Siletzia, you might expect higher pressures—and even better conditions for generating episodic tremor and slip!” But because it’s actually observed less often in and around Siletzia compared to regions without this highly resistive block, that implies there’s more to this phenomenon than high fluid pressure, he said. “As we say, necessary condition, but not sufficient.”